Glowing Plants: An Intro to Plant Engineering | 18
By following some spicy synthetic biology drama, we can understand a bit more about the basics of plant engineering 🌱
Glowing plants have long been the biologist's moonshot, something to behold on a black cinema screen, the star of a rhetorical question posed by high school teachers after explaining CRISPR to 12-year-olds. We promise a future full of glowing trees as a sustainable alternative to lamplights and beds of bioluminescent roses blooming down millionaire's hedges and walkways so quickly. Yet in reality, things are not so simple as this. Herein lies the decade-old problem of why it’s almost impossible to just “insert firefly genes into plants to make them glow.” And why genetic engineering takes so long and is so complicated.
After reading this article, you’ll hopefully have a deeper understanding of:
plant engineering (gene editing)
the basics of synbio and making plants glow
bioethics, regulation, and commercialization
Enter a tale of hope, (misguided?) techno-optimism, suicide, and why Kickstarter has BANNED all synthetic biology projects as rewards on their site.
We’ve made glowing plants before
Before you make the same mistake I did, DO NOT confuse bioluminescence with fluorescence.
Typically, when something “glows”1 under UV light, that’s fluorescence.
Wear white or neon to a blacklight party, and you’ll notice that your clothes begin to “glow”. That’s fluorescence. A lesser-known example of fluorescence being used in action involves GFP (aka. Green Fluorescent Protein) taken from jellyfish. Because GFP glows easily under UV light, the gene has been used for decades as a visual reporter–– aka. an easier way for humans to learn whether or not a gene is expressed. Fluorescent plants like these ones on the ISS are an example of plants that were engineered to include the GFP gene. When under UV exposure, they appear to emit light.
But how about actual glowing in the dark? We took a step towards this in 1986 when a team of scientists from UCSD created the first genetically modified bioluminescent plant. This time, no UV light was needed for the plant to glow.
A. The basics of bioluminescence
Luciferin and Luciferase (enzyme) (+Oxygen) = light💡
In nature, when luciferase comes into contact with luciferin (in the presence of oxygen), a chemical reaction takes place, and it results in a phenomenon called bioluminescence. Luciferin has stored energy, and luciferase is like the enzyme/catalyst that uses luciferin’s stored energy and turns it into the creation of light.
p.s. Luciferase is a generic term for the protein – evolutionarily speaking, different luciferases from different species have very little in common with each-other.
What the UCSD team did was transport the gene that could make luciferase (the light-emitting enzyme that’s in fireflies) into a plant so it integrated the process of luciferase production into its genome. Therefore when the plant was “watered” with luciferin, the plant would emit light! The two components reacted within the plant and produced light via that same bioluminescent chemical reaction mentioned above. So way back in 1986, we didn’t exactly have a plant that could glow by itself (aka. an auto-luminescent plant), but we did technically have a glowing plant.
The really huge breakthrough came when chloroplast engineering was used to create the first autoluminescent plant back in 2010. Chloroplast engineering means changing the DNA in.. you guessed it right–– the chloroplast (the parts that help plants make food from sunlight)! Scientists at SUNY found out a way to create plants that glowed in the dark without UV light or added luciferase but the problem was.. the glowing wasn’t very bright.
Nevertheless, in 2013, a man named Antony Evans decided enough technological progress had been made in the past 20 years–– it was time to turn this scientific theory into a raving commercial success. 🌟 Using the image of the glowing plant from the 1986 Science paper as the logo of their campaign, Antony and his team raised over $425,000 on Kickstarter, taking on the bold dream of producing glowing plants while educating people about synthetic biology–– stoking the hope of both amateurs and scientific professionals around the world.
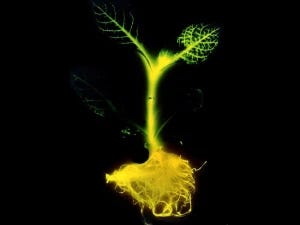
The project received support from many respected names in both the science and business world. These supporters of the project included George Church, Y-Combinator, Andressen-Horowitz, Peter Diamandis, and even Peter Thiel. Yet not a single glowing plant was ever shipped. What went wrong along the way?
Perhaps it was the Game Plan
DNA *synthetic operon ligate* -> Plasmid *heat shock insert* -> Agrobacterium (a type of bacteria) -> *infect plant genome* Plant genome is changed
The master plan was to insert bacteria’s entire lux operon (ALL 6 GENES) into a plant so it gained the capability to shine on its own. But how does “inserting glowing genes into a plant” actually work?
Inserting DNA from one living thing to another is called transformation, a process that’s pretty simple for bacteria and yeast (unicellular organisms) but a lot more complex when it comes to plants (multicellular organisms).
🔍 A nice summary of what they were trying to do was: add a set of glow genes called the "lux operon" from a bacteria called Vibrofischerii to the plant's genetic code. These genes were coordinated in a metabolic pathway that allowed the plant to produce all the necessary enzymes and molecules needed to make light, via bioluminescence.
How was this attempt different?
“Wouldn’t it be nice in the future if someone could just load up a tree’s genetic code, drag another app from a file and make it glow in the dark?”
- Omri Amirav-Drory, Founder of Genome Compiler
TAXA’s Glowing Plant project was using a brand-new software called Genome Compiler, co-founded by Omri Amirav-Drory. The software was a breakthrough technology in the field of Synthetic Biology back in 20132, and could, in theory, load up the existing sequences for any life form (ex. plants), and then allow the user to genetically manipulate them by inserting or taking out various genes. Genome Compiler could also perform basic validation and error detection for basic errors such as not having three codons for an amino acid or having a stop and a start codon in the wrong place.
Instead of inserting the glow genes into the chloroplast (like the chloroplast-engineering attempt back in 2010), Genome Compiler and Antony Evans intended to engineer the nucleus (nuclear expression) instead. Both chloroplast engineering and nuclear expression can change how the plant works but they target different parts of the plant cell. The problem with using chloroplast engineering for creating glowing plants was that they were:
Very dim, even when the strongest chloroplast promoter was used
A sickly light green color because the engineering of the chloroplast disrupted the production of chlorophyll, the pigment that gives plants their green color and allows them to carry out photosynthesis
There wasn’t any methodology yet for removing the antibiotic genes as the selectable markers (and it’s pretty obvious why releasing 1 million seeds of antibiotic plants into the wild is a bad idea3 😬)
The chloroplast-engineering approach was already patented, and this would have conflicted with the Glowing Plant company’s open-source approach
Nuclear expression seemed to hold much more promise for creating brighter glowing plants. But not everyone was impressed...
Critiques: A Lack of Actual Innovation?
Ginkgo Biowork’s current Creative Director Christina Agapakis published an article about the project entitled “Glowing Futures” in Scientific American back in 2013. Aside from scathing comments about the project’s overly techno-optimistic positioning, she states that when you looked closely at their methodology, there wasn’t actually too much of a difference at a technical level between what TAXA’s Glowing Plant project (backed by Genome Compiler) was trying to do and previous attempts.
So what's different between these past glowing plants and the plants proposed by Genome Compiler? At a technical level, besides using their software to optimize the genetic code for a different host organism (the Genome Compiler team is using Arabidopsis, not tobacco), not much. -Christina Agapakis, Creative Director of Ginkgo Bioworks, 2013
OG Synthetic Biologist Drew Endy also questioned how much light the plants would actually be able to emit, given the limitations on a plant’s ability to harvest energy from the Sun and convert it back into light.
“Never mind the genetic engineering involved — just what does the physics say about the feasibility of the project working out?” - Drew Endy
Despite the critiques and backlash, support for the Glowing Plant project was still strong during the early stages.
So Antony and his team started work on the first step of creating a glowing plant...
Making the VIP baggage: modified DNA & optimized lux operons
The Genome Compiler-backed Glowing Plant project involved modifying genes from the lux operon found in bioluminescent marine bacteria and optimizing them for expression in the plants.
The lux operon consists of several genes, including luxA, luxB, luxC, luxD, luxE, and luxG, which together encode for the enzymes and proteins responsible for bioluminescence. The idea was to create a synthetic version of the lux operon that would be functional in plants so that the plant gained the ability to naturally produce the enzymes and cofactors necessary for bioluminescence like bioluminescent bacteria naturally did.
It was necessary that they inserted the entire lux operon because it ensured that all 6 of the necessary components for bioluminescence were present. (ex. the luciferase enzyme that catalyzes the reaction to produce light + other proteins that are necessary for the reaction to occur efficiently). By introducing the bacteria’s entire lux operon, scientists could take advantage of how nature had optimized this process of bioluminescence through millions of years of evolution, and make it more likely that the genes would be successfully expressed and therefore result in more energy-efficient bioluminescence in plants. If only one or two of the genes were introduced, the pathway might not function properly (due to the missing components), and the plant bioluminescence would likely be very weak or nonexistent.
This is what happened to TAXA’s Glowing Plant project. The full lux construct was never transformed. They were able to synthesize a whole lux construct but weren’t ever able to insert all 6 genes.
What makes up the Lux Operon: (not important but it's good for you to visualize this)
1. luxA - encodes for the alpha subunit of luciferase, the enzyme that catalyzes the light-producing reaction
2. luxB - encodes for the beta subunit of luciferase
3. luxC - encodes for the enzyme responsible for producing the luciferin substrate required for the light reaction
4. luxD - encodes for the enzyme responsible for recycling luciferin 5. luxE - encodes for a fatty acid reductase that produces a long-chain aldehyde required for the light reaction
6. luxG - encodes for an enzyme that modifies the long-chain aldehyde to make it more reactive with luciferase.
The Glowing Plant was most successful at the Codon Optimization strategy:
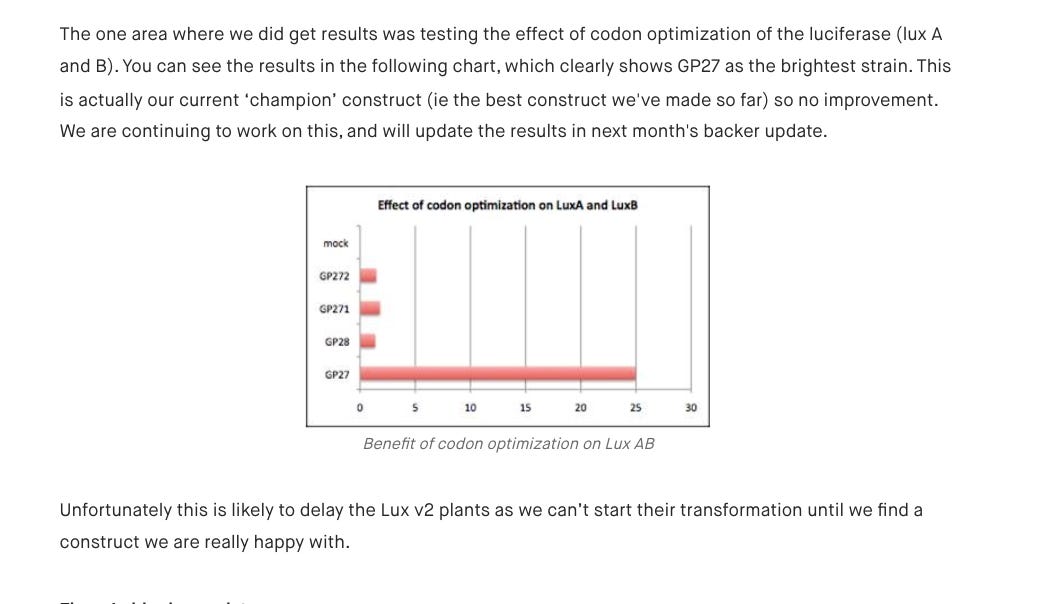
What is Codon Optimization?
You can use different combinations of codons to make the same protein. Plants and bacteria prefer different codons. Codon optimization is switching out the codons so that what is used to create the amino acids that make up the protein are the codons preferred by the organism.
Since there are 64 codons (triplet sequences of nucleotides) but only 20 amino acids, multiple codons can code for the same amino acid. And as amino acids are the building blocks of proteins, you can mix and match codons but still get the same protein. Yep! The same protein can be encoded by different sets of codons, as long as they all specify the same amino acids in the correct order.
🔍 The same amino acid can be encoded by different sets of codons.
This is important because different organisms have different preferences for which codons they use more frequently. By using codon optimization for the bioluminescence lux operon, the plant can create the proteins needed for bioluminescence using the codons that work best for them–– resulting in an improved overall function (brighter, longer-lasting glow).
Once you have designed and compiled the optimized genetic code, however, you’ve got to print it and insert it into existing cells.
That’s where Cambrian Genomics comes in. Founded by IGEM whiz kid Austen Heinz, Cambrian Genomics was a new breakthrough DNA synthesis company boasting a drastically lower error rate and price that came from their pioneered method called “laser-based DNA synthesis”, a technique that leveraged DNA laser printing to create synthetic DNA molecules with high precision and efficiency.
Austen was another starry-eyed silicon valley founder who wanted to change the world, so it makes sense that he was attracted to the flashy Glowing Plant venture.. though of course, neither one knew how the story would end. They, along with Genome Compiler were the biggest early- supporters of the Glowing Plant project and were involved in synthesizing the specific genes responsible for the desired glowing trait in the plant–– utilizing their expertise in DNA synthesis to give expert advice to TAXA as they created their custom DNA sequences.
Plasmids = DNA carriers
Alright. So we’ve optimized the glow system and adapted it to fit into plants, then printed it out. Now how do we actually insert the optimized lux operon we made using modified DNA into the plant?
Answer: Bacteria accept new DNA code relatively easily if carried by a plasmid. A plasmid is a small circle of DNA with its own origin of replication, which allows it to replicate independently (completely apart) from the bacterial chromosomes. Think of plasmids as delivery trucks for the DNA, vehicles, or “vectors” as almost everyone calls them. Whatever suits your preference. The point is, they’re important because plasmids are in charge of carrying the DNA and introducing foreign genetic material into a host organism, like bacteria, yeast, or animal/human cells.
Key parts of a plasmid include:
Origin of replication (ori): This is a specific DNA sequence that allows the plasmid to replicate independently from the host. The origin of replication determines the plasmid's “copy number” (how many copies of the plasmid are present in the cell). If plasmids did not have their own origin of replication, the replication process would be slower or even fail because the plasmids would have to compete for resources (as bacterial replication machinery is optimized for the replication of the bacterial chromosome, and it may not have enough resources like energy, necessary proteins or enzymes to efficiently replicate plasmids as well).
Selectable markers: These are genes inserted to help researchers identify which cells have successfully integrated the plasmid vs. cells that haven’t. These selectable markers give special traits like antibiotic resistance to modified DNA. Antibiotics are usually used to exterminate cells that don’t adopt the genes. If the gene is incorporated successfully, the plant will be antibiotic-resistant due to the added antibiotic-resistant reporter genes. Methodology-wise, this works out pretty well as the genes that do not have the inserted plasmid (and therefore the selectable marker) are, upon exposure to antibiotics, exterminated.
Multiple cloning site (MCS): This is what helps us copy-paste the foreign DNA into the plasmid. Before putting the lux genes into the circular DNA, scientists add special markers (restriction sites) to the ends of the lux genes. They then use special scissors (restriction enzymes) to cut the lux genes and plasmid DNA, creating matching ends. Next, they mix the lux genes and plasmid DNA with a sort of "glue" called DNA ligase. This glue joins the matching ends together, creating a new circular DNA (recombinant plasmid) with the lux genes inside. All this was enabled and brought to you by the existence of the Multiple cloning site (MCS) and its restriction recognition sites.
Promoters and other regulatory elements: Promoters are specific DNA sequences that act as control regions for gene expression. They help initiate the process of transcription, which is the first step in producing a protein from a gene. By changing the promoter sequence or using different promoters, scientists can control the expression levels of genes in its target host. Testing different promoters allows researchers to determine which ones result in the best expression levels for a specific application or desired outcome.
Gene circuits and Plasmids
DNA *synthetic operon ligate* -> Plasmid *heat shock insert* -> Agrobacterium (a type of bacteria) -> *infect plant genome* Arabidiopsis Plant genome
Alright, this is something I was also confused by at some point: a plasmid is not a gene circuit itself, but it can contain gene circuits. Assembling your gene will be like assembling a circuit and involve components like promoters, repressors, activators, and other genetic elements that control gene expression. Engineering these circuits accordingly can be used to create complex behaviors–– with cells responding to certain signals or producing specific outputs.
The promoter and terminator mark the start and the end of transcription, and a ribosome-binding site (RBS) is responsible for the correct initiation of translation. As suggested by the name, ribosome-binding sites (RBS) are sequences where the ribosomes - the translation machinery - attach in preparation for making the protein. Without an RBS sequence, the ribosomes would not be able to position themselves properly onto the mRNA transcript and, as a result, the translation of the mRNA into protein would completely fail.
When we say a gene circuit is "assembled on a plasmid," we mean that the gene circuit is incorporated into the plasmid's DNA. This is typically done using techniques such as restriction enzyme digestion (restriction enzymes cut DNA molecules at specific recognition sites) and ligation (Ligation is the process of joining two DNA molecules together using an enzyme called DNA ligase). The gene circuit's DNA is inserted into the plasmid, creating a recombinant plasmid containing the gene circuit.
Agrobacterium Infects Plants
DNA *synthetic operon ligate* -> Plasmid *heat shock insert* -> Agrobacterium (a type of bacteria) -> *infect plant genome* Arabidiopsis Plant genome
The plasmid then gets inserted into a bacteria (ex. e.coli). For plants, the go-to bacteria is Agrobacterium tumefaciens, a soil bacterium that naturally infects plants. Agrobacterium tumefaciens is considered the most widely used and efficient method for plant genetic engineering as the bacteria can be used to deliver the plasmid into the plant's cells. Once inside the plant's cells, the plasmid integrates into the plant's genome, allowing the genes to be expressed and produce light.
Heat Shock
To insert plasmids into bacteria, the Glowing Plant project in particular used the heat shock method (one of the most widely used methods). Rapidly heating and cooling the bacteria creates cracks that allow bacteria to accept the plasmid. After, the cells are usually incubated at a lower temperature to allow them to recover and express the newly-introduced genes.
Heat shock method: Insert DNA into agrobacterium via heat shock (heating and cooling). Rapidly heating and cooling the bacteria creates cracks that allow the bacteria to accept the plasmid. The heat shock method is widely used for introducing plasmids into competent bacterial cells (cells that are prepared to take up foreign DNA).
In this method, a mixture of competent bacteria and plasmid DNA is subjected to a brief heat shock (typically at 42°C for 30-90 seconds), followed by rapid cooling on ice. The sudden temperature change creates a transient increase in the permeability of the bacterial cell membrane, allowing the plasmid DNA to enter the cell. After the heat shock, the bacteria are usually incubated in a recovery medium to allow them to express the plasmid-encoded genes, such as antibiotic resistance markers, before being plated on selective agar plates.
🔥→🌿 So plasmids containing the lux operon are inserted into Agrobacterium via heat shock.
? How to infect plants via Agrobacterium
Flowers of the plant are dipped into a solution containing the transformed bacteria (agrobacterium). The bacteria inject the DNA into the cell nucleus of the flowers which pass it onto their seeds that the Glowing Plant can then grow until they (hopefully) glow!
The Downsides of dear Agrobacterium
It’s a plant pest :( Meaning that anything created with agrobacterium will be heavily regulated by the FDA. Because of this, the Glowing Plant team only used Agrobacterium during the designing of the luciferase genetic circuit.
When they actually had to mass-produce the plants for public consumption, the TAXA’s Glowing Plant project bombarded the genes into plant cells using a ballistics-powered device called a “gene gun”. This was more complicated, due to the risk that the gene sequence would get scrambled, but at least the results would be unregulated by the USDA and therefore suitable for release.
Gene Gun WHAATT??
GENE GUNNNNN: Coat gold nanoparticles with plasmids that contain the foreign DNA and bombard the plant. All hail the power of strong mechanical force!
Admittedly, when it comes to introducing the modified DNA and plasmids into plants, a gene gun sounds way cooler than a bacterial delivery system.
A gene gun, or “biolistic particle delivery system,” is a device used for introducing foreign DNA into cells by propelling DNA-coated microprojectiles into target tissues. This method is also known as “bombardment”. The gene-gun method is most commonly applied when doing plant transformation but can also be used for other organisms, such as fungi, algae, and animal cells. This is typically done by coating small metal particles (such as gold or tungsten) with the desired DNA, and then accelerating these particles at ultra-high speeds into the target cells using the gene gun.
When the gene gun is then fired, it releases helium pressure and propels the microprojectiles into the target tissue. The particles penetrate the cells, delivering the foreign DNA into the cell nucleus. This high speed of the particles is what allows them to penetrate the cell walls and membranes, so the genetic material they carry can be integrated into the host genome. After transformation, the tissue is allowed to recover and express genes, and cells that have successfully integrated the foreign DNA are selected using a “selectable marker”, such as antibiotic resistance, and allowed to grow. (as discussed earlier in the plasmid section).
In the case of plant transformation, whole plants are regenerated from the transformed cells or tissue, and the regenerated plants are analyzed for the presence and expression of the introduced genes.
Pros of a gene gun:
Broader applicability: The gene gun method can be used to transform a wider range of plant species compared to Agrobacterium-mediated transformation (limited by its natural host range).
Loopholing Regulatory concerns: Because Agrobacterium is a plant pathogen that can cause diseases, such as crown gall disease, there are many regulatory concerns and hurdles surrounding its use in genetic engineering. By using a gene gun, the Glowing Plant team was able to loophole regulation as this method does not involve the introduction of foreign DNA from a pathogen. This is especially important when working with genetically modified organisms (GMOs) intended for release into the environment or commercialization.
While the gene gun method has its advantages, it also has some drawbacks, such as the potential for causing more damage to plant cells and tissues during the transformation process and the possibility of introducing multiple copies of the desired genes or fragmented DNA into the plant genome. Nonetheless, for the Glowing Plant Project, the benefits of using a gene gun outweighed the limitations, making it a suitable choice for the transformation of the target plant species.
"We generally use the biolistic method for stable transformation, though we do have agro-bacterium methods available in order to prototype more quickly (which is the version we've demoed previously). While the biolistic method is slower and more expensive to generate a single plant, it's key advantage is that - if care is chosen with DNA parts used - the final product is immediately free for sale and distribution in the United States without requiring regulatory review. This saves years and millions of dollars from the budget for getting the product to market and made this whole kickstarter possible."
- Antony Evans, Glowing Plant Kickstarter Update
Something to Target: Plants
I used to ask "Why would they try to make tobacco plants glow out of all things?"
Well, to be fair, the Glowing Plant project actually started with Arabidopsis thaliana (thale watercress) but made a decision to switch to tobacco. Arabidopsis was first chosen because of its small genome size, but the two main issues with Arabidopsis were the low transformation efficiency and the challenges people under short daylight conditions would have when growing them at home.
They then decide to switch to tobacco because it had a simple genetic code–– making it easier to insert a plasmid with the foreign DNA (aka. luciferase) into the plant's genome. Ofcourse, there are other key reasons why tobacco is such a suitable target such as:
Well-established transformation protocols: Tobacco plants have well-established methods for genetic transformation using Agrobacterium-mediated gene transfer or the gene gun approach. These protocols have been refined over time, making it relatively easy to introduce foreign genes into tobacco plants.
Rapid growth and short generation time: Tobacco plants grow quickly and have a relatively short generation time (around 12-16 weeks from seed to seed), allowing researchers to observe the effects of genetic modifications in a short period of time.
Large size and abundant tissue: Tobacco plants produce a large amount of biomass and have sizable leaves, which are particularly useful for experiments that require a large amount of plant tissue or when testing the expression of recombinant proteins.
Susceptibility to Agrobacterium: Tobacco plants are naturally susceptible to Agrobacterium tumefaciens infection, making them an ideal host for Agrobacterium-mediated genetic transformation, which is a widely used method for introducing foreign genes into plant cells.
How it all went and worked together
How did they make sure the plants were glowing as brightly as possible? What sorts of things were they changing and what did they experiment with?
Throughout the entire process, the Glowing Plant team relied on transient assays.
In a transient assay, scientists introduce the gene or protein they want to study into cells, but just for a short period of time. It's like putting a small, temporary sticker on something to see how it looks or behaves. This allows scientists to observe what happens when the gene or protein is present in the cells without making any permanent changes.
By doing this, scientists can quickly see if the gene or protein has any noticeable effects on the cells or if it interacts with other molecules. It's sort of like taking a sample or a small taste of a dish to see if it has the flavors and ingredients you want.
Pros: Transient assays quick and useful for easy and efficient experiments. They allow researchers to quickly introduce and express a gene of interest in target cells, bypassing the time-consuming process of generating stable cell lines.
““Cons”: Transient assays don't last long because the introduced gene or construct is not stably integrated into the organism's genome. As a result, the gene or construct can be lost during cell division or simply degraded over time, so it only expresses the gene temporarily. Additionally, since the introduced DNA doesn't become a permanent part of the genome, it is not inherited by the offspring of the organism.
Promoter journeys and adventures
Promoters are specific DNA sequences that help initiate the process of transcription, which is the first step in producing a protein from a gene. By changing the promoter sequence or using different promoters, scientists can control the expression levels of genes in its target host.
To improve the luminosity of plants it was essential that they found new promoters which were highly expressive. Candidate promoters were fused to the firefly luciferase gene and the relative strength of each promoter was tested by transient assays. TAXA’s Glowing Plant made versions using several different promotes and several combinatorial designs which combined promoters in case of gene silencing.
When more is actually less
There were some shocking results when they identified a promoter that demonstrated a 100x performance compared to other promoters. They expected it to lead to the brightest plants, but upon further testing, they realized that it didn’t. To their surprise, the overexpression of any group of genes lead to a negative effect on the activity. And upon further investigation, they came up with several possible explanations to understand the main cause of the negative results:
Silencing in plant cells, which means the cell shuts down the promoter activity or degrades the RNA of the highly expressed genes to reduce the negative effects resulting from high expression
High levels of lux genes are toxic to the plant cell
An unidentified mutation in the promoter means none of those were working correctly
Selectable marker Switcheroo
So you remember how an easy way to tell if a plant has successfully incorporated a gene is if it doesn’t die from the antibiotics because of the antibiotic-resistant reporter genes?
It’s a clever strategy but it resulted in a low-efficiency problem for the team–– especially when compounded with the many difficulties they faced inserting an intact full construct containing all the genes. So, TAXA worked on a new selectable marker gene cassette that did not need to be crossed out (more info here).
The new selectable marker was based on a different conformation of amino acids. Each amino acid can exist in two different types, L-type and D-type, and depending on the species, one type can be used while the other is toxic. The researchers found a gene that could convert the toxic type to the beneficial type in plants, which could rescue the plant from growing on a media supplemented with the toxic form of amino acid. To confirm that the new selectable marker could be used in their experiments, the researchers grew tobacco and Arabidopsis plants on media containing the toxic form of amino acid and confirmed that it was indeed toxic to the plants. 🎉
The new selectable marker gene cassette that they developed was thus, more efficient and did not have the environmental concerns associated with hygromycin (the antibiotic).
In the end, they were able to get 4 constructs, though none of them shipped.
Fluc v1.0 - very dim (only just visible in a pitch dark room) and required luciferin substrate.
Fluc v2.0 - 10 times brighter than v1.0 (based on luminometer readings) also requires substrate.
Lux v1.0 - auto-luminescent, transients indicate luminosity somewhat similar to Fluc v1.0 though unclear what that will mean with full grown plants - first plants currently being regenerated following bombardment
Last Update --------> "We are still in the process of regenerating the plant. The first batch of regenerants didn't glow stably, although we did find one weird plant which was glowing a little but which then stopped glowing which was a very strange and unexplained result."
Lux v2.0 - auto-luminescent
Last Update --------> "We discussed the germination problems in the last update.Our hypothesis about anther sterility was correct and now we have nicely germinated T2 lines growing in our growth tent. These plants will be tested for luminosity and marker presence next week. Once we find one that glows nicely without a marker sequence, we will be ready to grow that one to make it's own seeds and ship out like the Fluc v1 plants - so this is back on track."
Why TAXA’s Glowing Plant didn't work out:
As it happened, they never got all six genes into the plant. It's still unclear whether this was caused by the toxicity of the genes or challenges with getting such a large construct inserted correctly. Their primary hypothesis was the latter. TAXA’s Glowing Plant project was unable to get ahold of luxC, the sixth and last gene necessary for the plant's complete genetic modification.
"Assuming we succeed in doing that [getting luxC], I think at this stage it's still possible," - Antony Evans, Glowing Plant Founder
A doomed cause?
The team underestimated the difficulties they’d face with plant engineering. They knew a dimly glowing tobacco plant had been made before, in 2010, but the scientist who carried out that work, Alexander Krichevsky, says it took him three years leading a lab at a well-equipped university, SUNY Stony Brook, to do it. The organism doesn’t want to waste its energy on an extraneous process like glowing and is liable to undertake complex countermeasures. "BURDEN" occurs when tons of new genes are added to a cell. The glowing genes become a hassle for the cell because they require energy to function–– energy that’s taken away from other important functions like growth and metabolism.
“Even if six genes are added correctly (itself a daunting challenge), the plant will furiously attempt to silence or extinguish them” - Krichevsky.
As a final kicker, luciferin can potentially be toxic to plants if it accumulates in high concentrations, as it can interfere with normal metabolic processes and cause oxidative stress. With all of these obstacles blocking the expression of bright bioluminescence without killing a plant, creating glowing flowers remained an unsolved challenge.
"Although bacterial bioluminescence genes can be targeted to plastids to engineer autoluminescence, it is technically cumbersome and fails to produce sufficient light.”
Self-admittedly, they were years too early working on this technology. Christina Agapakis’s (current creative director of Ginkgo Bioworks) comments on their procedures as a critique of the synthetic biology industry’s problems at large.
So is it the same or is it different? While aggressively selling themselves as something new and something inherently moral and democratic, Genome Compiler is unfortunately acting out a very familiar story. They are a for-profit corporation trying to wedge themselves into a crowded market of DNA-based services that has many viable open-source alternatives, exploiting the ideas, work, and enthusiasm of idealistic people excited about the potential of synthetic biology, overpromising and overhyping the potential of the technology in the short term in order to market their derivative, proprietary software tools and themselves as leaders in the future of the field.
…Will we someday have glowing plants lighting our streets, "solving" the problem of electricity use for lighting? Probably not. Will corporations continue to try and exploit others and their ideas to make a profit? Unfortunately yes. - Christina Agapakis, 2013
Much other controversy surrounded TAXA Biotechnology’s attempt to create a Glowing Plant. Subject to much scrutiny from biologists, bioethicists, and Kickstarter backers, many media outlets released reports of Taxa’s failure to deliver on its promise due to reasons spanning from a lack of preparation to sheer bad luck.
Remember the guy who founded Cambrian Genomics and was one of TAXA’s earliest supporters? He killed himself in May 24, 2015 due to mental health issues that come from not being able to properly handle the pressures of being a founder while going through some tough media-related drama.4
Something to protect: A dream
While many prominent and respected figures were irked by TAXA’s story, many were also touched by their earnest attempt to create Glowing Plants.
Many have mentioned how Antony’s frequent and scientifically-intensive updates helped them learn about synthetic biology. Though the team constantly faced problems, Antony never once hid behind a lack of communication and transparently communicated the science behind what the team was doing. Due to this, a sizeable amount of the Kickstarter supporters were still appeased by Taxa’s efforts, citing that their integrity and daring to start this ambitious project was inspiring enough for them to invest their money to be a contributing part of the story regardless of how it turned out.
And that’s what’s important, isn’t it? Glowing plants have long been the biologist’s moonshot. While TAXA failed its goal to ship glowing plants, it was still (even at its worst) a company that tried its best to bring forth an interesting future. They also ended up posting 69!! updates about the Glowing Plant, educating many (including myself!) about the many trials and tribulations of plant engineering in the context of a startup. Finally, while they didn’t ship Glowing Plants, they ended up hey ended up finally selling a product called “Orbella Fragrant Moss”, bioengineered moss that smelled of patchouli and other fragrances. Their packaging was even completely biodegradable and made out of mushroom foam designed by Ecovative, a New York-based biomaterials company (that’s also one of my fav synbio companies).
TAXA was a failed startup for plant engineering and the creation of Glowing Plants, but it was able to inspire a lot of people with a story. A triumphant future of open source synbio and doing "something that people on the street would consider science fiction”, as the company put it.
Evan’s last Kickstarter update ends with an apology, “I’m sorry that we’ve let you down on delivering the Glowing Plant,” he writes. “I hope though that despite that failure the project can still leave a positive legacy in inspiring people to learn more about synthetic biology and its benefits — and hopefully one day someone does finally make a Glowing Plant.”
But perhaps the damage to the image of synbio was irreparable. After the successful Glowing Plant campaign, the Kickstarter site has added a new term to its guidelines stating that "projects cannot offer genetically modified organisms as a reward”, effectively banning the use of GMOs as rewards for any future projects.
Moonshots are a double-edged sword, but along with progress, comes failure. We must learn to put in the Ls (and a whole bunch of them) if we want to truly change the world. The Glowing Plant transformed into another notch on the history of glowing plants timeline, but maybe every brick counts, and this helped lead the way for other companies and individuals to try their hand at bringing forth the future of Avatar lights and beautiful glowing flowers.
Almost but not quite: Plant Nanobionics
Another “almost!” for the Glowing Plant saga
At MIT, a team led by Professor Michael S. Strano worked on developing a “nanobionic” glowing plant. They crafted nanoparticles that could travel to specific destinations within plants and deliver the luciferins and enzymes needed for bioluminescence.
These nanobionic plants could do crazy things. Previously, they’d been able to design plants that could detect explosives and communicate that information to a smartphone, as well as plants that could monitor drought conditions. Strano’s group’s goal was to engineer plants to the point where they could take over many of the functions performed by electrical devices.
Okay, how does “Plant Nanobionics” work?
🔍 Embed plants with tiny nano-particles and you get plant nanobionics.
The researchers had this idea of packaging the three essential components for bioluminescence—luciferase, luciferin, and coenzyme A—into separate nanoparticles.
Then, they found a way to sneak these nanoparticles into plant leaves.
Q: How?
A: By soaking the plants in a nanoparticle solution and exposing them to high pressure, allowing the nanoparticles to enter the leaves through small pores called stomata.
Imagine the mesophyll—an inner layer of the leaf—as a sort of meeting spot for these nanoparticles. The larger ones carrying luciferin and coenzyme A hang out in the extracellular space, while the smaller ones carrying luciferase slip inside the mesophyll cells. The nanoparticles gradually released luciferin, which entered the plant cells where the luciferase enzyme facilitated the bioluminescent reaction. When they all meet, the nanoparticles release their goods, creating a fantastic glow in the plants.
At first, Strano’s MIT plant engineers got the plants to glow for around 45 minutes. But, being the ambitious researchers they were, they pushed their limits and managed to extend the plant’s glow to 3.5 hours. Super impressive. The glow wasn't quite bright enough to read by (the light generated by the plants was still about one-thousandth of the amount required for reading), but hey, it was a start! The method could be used on any type of plant, and they demonstrated proof of concept with arugula, kale, and spinach, in addition to watercress.
Grand Finale: A Successful Glowing Plant!!
Credits to: Caffeic Acid and LightBio
p.s. caffeic acid has no relation to caffeine!
When worlds meet
A Cool Synbio Crossover:
Kevin Wood,one of the main authors of the 1986 paper where they first made plants glow, and Dr. Omri Amirav-Drory, founder of Genome Compiler and one of the co-founders of Glowing Plant (remember him?), are now both working on a company called light.bio! Unlike previous genetically engineered glowing plants, which used bioluminescent bacteria or firefly DNA, light.bio ‘s plants make use of the DNA of bioluminescent fungi. 🍄
They discovered glowing fungi that could create luciferin using a compound called caffeic acid. Two enzymes in the fungi work to transform caffeic acid into luciferin; and a third enzyme oxidized this precursor to produce a photon. A fourth enzyme converted the molecule back to caffeic acid, which could then be recycled through the same process.
The Glowing Fungi genes: Neonothopanus nambi bioluminescence genes: nnluz (luciferase), nnhisps (hispidin synthase), nnh3h (hispidin-3-hydroxylase) and nncph (caffeoyl pyruvate hydrolase)
Now see - caffeic acid is found in all plants. It's a key ingredient in the creation of lignin, a wood polymer that gives plant cell walls rigidity and strength. The team reasoned that it might, therefore, be possible to genetically engineer plants to reallocate some of their caffeic acid to the biosynthesis of luciferin (as seen in bioluminescent fungi). The scientists only had to add just four extra ‘bioluminescence’ genes from the bioluminescent fungi, in order to create transgenic plants with a new ‘Caffeic Acid Cycle’.
They spliced their tobacco plants with four fungus genes associated with bioluminescence and carefully cultivated them. The introduction of these new genes enabled the tobacco plants to produce the necessary enzymes for bioluminescence, allowing them to glow. And they found that the plants glowed with a light visible to the naked eye from seedling to maturity - without any apparent cost to the health of the plant. "The overall phenotype, chlorophyll and carotenoid content, flowering time and seed germination did not differ from wild-type tobacco in the greenhouse, with the exception of a 12 percent increase in median height of transgenic plants,"
This suggests that, unlike the expression of bacterial bioluminescence, expression of caffeic acid cycle is not toxic in plants… and maybe it’s even somehow beneficial?
They found that younger parts of the plant glowed most brightly, with the flowers growing brightest of all–– producing around a billion photons per minute. That's not nearly enough to read by, but it is bright enough to be clearly visible.
According to the researchers, it’s also around 10 times brighter than other genetically engineered glowing plants!
This is all still a pretty difficult undertaking as the light bio team are literally introducing genes from a whole other kingdom and engineering fungal bioluminescence genes into a plant’s nuclear genome. So these glowing flowers aren’t able for shipping yet… BUT with the aid of Ginkgo Bioworks, hopefully they will be soon.
https://twitter.com/FeyScientist/status/1514017180274200578?s=20
https://twitter.com/Ginkgo/status/1514715201886588930?s=20
p.s Why name it the firefly petunia? Maybe this will confuse people about how it works xD
Summary:
The Glowing Plant project may have been a failure, but it was a crucial step forward in the history of glowing plants. Every attempt at innovation and progress is a brick added to the foundation of our future. And even though TAXA may not have succeeded, they paved the way for other companies and individuals to continue experimenting and pushing the boundaries of plant engineering.
So.. Long live Glowing Plants!
FAQ:
Why didn’t they just use CRISPR?
Where is Antony Evans (founder of Glowing Plant) now?
COO at Wild Earth (a dog food synbio company)
an awesome biotech startup using cultured proteins to develop clean, high-quality pet foods for the $30 billion pet food market.
I want to buy a glowing plant!
You can buy one
The company Aglaé developed a biodegradable elixir that is absorbed by the plants, causing them to emit a glowing light when placed under a black light.
Speaking to Design Boom, the company said: “This technology enhances the beauty of flowers and foliage. Thanks to it, they display a new aesthetic by revealing magnificent veins and coloured shades, from purple to blue/green.”
While the company is staying mum on exactly what’s in the serum, the team says it’s not harmful to the environment.
You can DIY and make your own fluorescent ones
I love glowing flowers a lot so I made some for my boyfriend during Valentines day cause he really likes the Avatar series.
Sources
"Peter Thiel invests in genetically modified glowing plants" - The Guardian, May 2013 Link: https://www.theguardian.com/environment/2013/may/09/peter-thiel-glowing-plants
https://blogs.scientificamerican.com/oscillator/glowing-futures/
https://www.smithsonianmag.com/smithsonian-institution/these-glowing-plants-could-one-day-light-homes-180972238/
https://www.kickstarter.com/projects/antonyevans/glowing-plants-natural-lighting-with-no-electricity
https://www.theatlantic.com/science/archive/2017/04/whatever-happened-to-the-glowing-plant-kickstarter/523551/
https://en.wikipedia.org/wiki/Glowing_Plant_project
https://www.technologyreview.com/2016/07/15/158184/why-kickstarters-glowing-plant-left-backers-in-the-dark/
https://www.theguardian.com/environment/true-north/2013/jun/06/kickstarter-money-glow-in-the-dark-plants
https://www.cnet.com/culture/kickstarter-bans-genetically-engineered-rewards/
Fluorescence is a phenomenon in which a substance absorbs light at a specific wavelength (often UV light) and then emits light at a longer wavelength, typically in the visible range. This emitted light can create the appearance of glowing or fluorescence, but it is not the same as simply glowing under UV light.
Since then, alternative softwares to Genome Compiler have emerged–– such as:
Benchling: It is a comprehensive platform with a wide range of features and strong collaboration capabilities. It offers a user-friendly interface and supports various molecular biology applications like designing and manipulating DNA sequences, managing projects, and collaborating with team members.
SnapGene: SnapGene is known for its intuitive interface and easy-to-use DNA visualization tools. It is popular for its ability to simulate and plan molecular cloning experiments effectively. It offers features for DNA sequence design, cloning, PCR primer design, and virtual cloning simulations.
Geneious: Geneious is a versatile software with a broad range of bioinformatics capabilities beyond DNA sequence design. It is suitable for users who require advanced analysis and molecular biology workflows. It includes features for DNA sequence design, gene annotation, primer design, sequence alignment, and more.
ApE (A plasmid Editor): ApE is a simple, free, and lightweight software specifically designed for plasmid editing. It is popular among researchers working with plasmid-based genetic engineering. It offers a straightforward interface for manipulating DNA sequences, performing restriction analysis, and designing primers.
Releasing pest-resistant plants may seem like a good idea at first glance, as it can reduce the use of pesticides and increase crop yields. However, it has several negative consequences that make it a bad idea.
Firstly, pest-resistant plants can harm the environment by reducing biodiversity. When a single type of plant is grown extensively, it can lead to the loss of other plant species and disrupt local ecosystems. Secondly, pest-resistant plants can also create new pests that are resistant to the plant's resistance. This can lead to a cycle of constantly developing new pest-resistant plants and pests. Lastly, pest-resistant plants can also harm human health. The use of genetic modification to create pest-resistant plants can lead to unintended consequences, such as the creation of new allergens or toxins.
In summary, releasing pest-resistant plants may seem like a quick fix to pest problems, but it can have long-term negative consequences on the environment and human health.
Austen Heinz’s story is sooo interesting, I have to write a quick blog post about it at some point. TLDR; At a presentation in November 2014, Heinz took the stage and started talking about Sweet Peach, a company that was working on probiotics for vaginas, and in which Heinz owned a 10% share. The media storm presented Heinz as sexist and juvenile: “Male startup founders want to enable women to change the way their vaginas smell.”
The media backlash and hate affected Heinz’s ability to raise more money for Cambrian Genomics. He had already been suffering from mental health issues so this situation exacerbated it. “It was the bad press surrounding this ten minute talk which led to repeated online mobbing and vilification of Heinz, which then led to his loss of investors, which then led to a major depression, which then led to his suicide. “
Amazing article. Great job teaching the science while also telling a fascinating story.